A Comparative Analysis of Carbon Capture Technologies
- Hüsnü Tolga Eyyuboğlu
- Jan 27
- 5 min read
Updated: Mar 5
As the global community continues to battle the adverse effects of climate change, carbon capture technologies (CCTs) have emerged as a pivotal tool in mitigating greenhouse gas emissions. These technologies offer the potential to significantly reduce the amount of carbon dioxide (CO2) released into the atmosphere from industrial processes, power generation, and other sources. With the growing emphasis on achieving net-zero emissions, understanding the different carbon capture technologies available, their efficiencies, costs, and scalability is critical for industries, policymakers, and researchers.
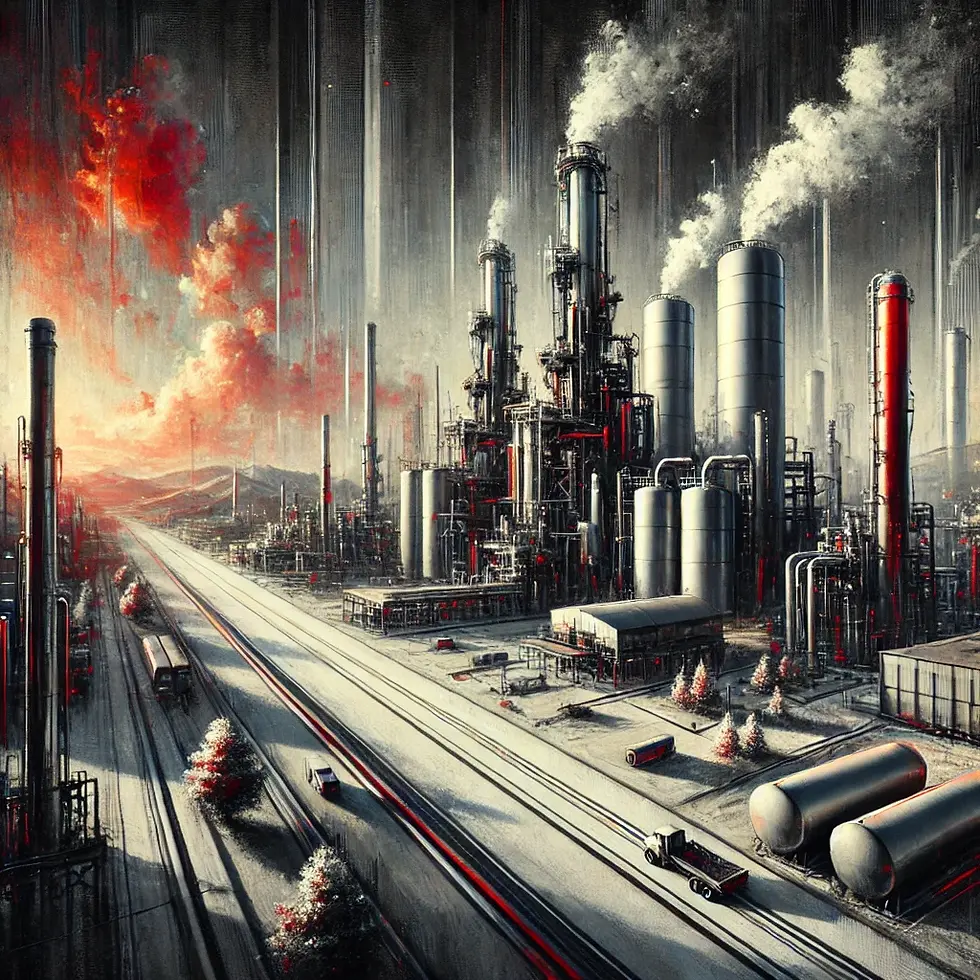
This article provides a comprehensive comparative analysis of the major carbon capture technologies, including their mechanisms, advantages, limitations, and potential applications. The focus will be on three main categories: post-combustion capture, pre-combustion capture, and oxy-fuel combustion, along with emerging innovations in the field.
Overview of Carbon Capture Technologies
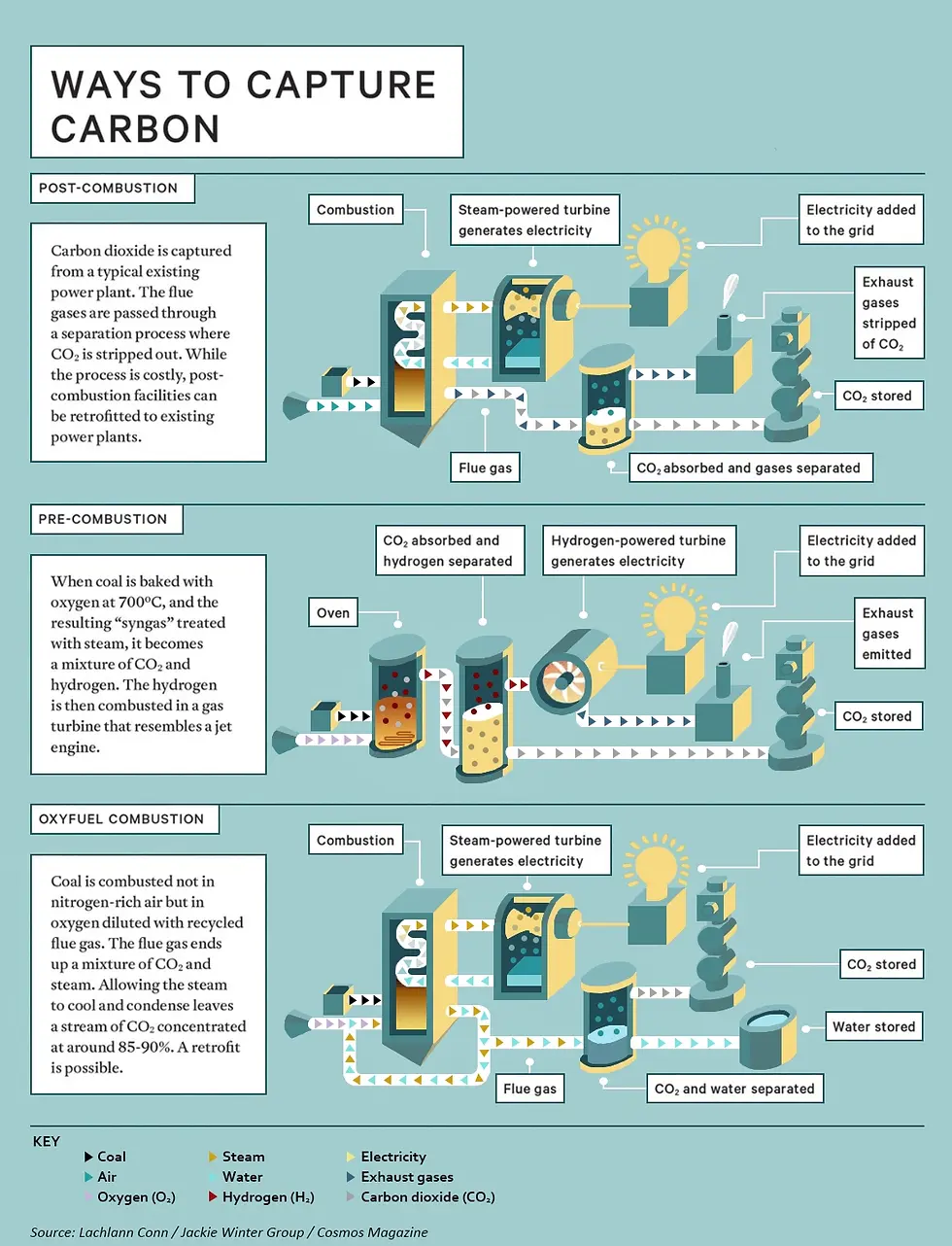
1. Post-Combustion Capture
Post-combustion capture is the most widely used and commercially mature method for capturing CO2. This technology involves extracting CO2 from the flue gases produced after fossil fuels are burned.

Mechanism:
Flue gases, typically containing 10-15% CO2, are passed through a solvent or sorbent that selectively absorbs CO2.
The CO2-rich solvent is then heated to release the captured CO2, which can be compressed for storage or utilization.
Advantages:
Can be retrofitted to existing power plants and industrial facilities.
Relatively mature technology with proven efficacy.
Compatible with various fuel types, including coal, natural gas, and biomass.
Limitations:
High energy requirements for solvent regeneration (parasitic energy loss).
Potential for solvent degradation and emissions of secondary pollutants.
High operational costs.
Applications:
Power plants, cement factories, and steel manufacturing units.
2. Pre-Combustion Capture
Pre-combustion capture involves removing CO2 before the combustion process by converting fossil fuels into a mixture of hydrogen and CO2.
Mechanism:
Fuels are subjected to gasification or reforming processes, producing a synthesis gas (syngas) composed of hydrogen and CO2.
The CO2 is separated using physical or chemical absorbents.
Advantages:
High CO2 concentration in the syngas stream simplifies separation.
Produces hydrogen, a clean energy carrier, as a byproduct.
Lower energy consumption compared to post-combustion methods.
Limitations:
Complex infrastructure requirements.
Limited applicability to specific facilities, such as Integrated Gasification Combined Cycle (IGCC) plants.
High initial capital costs.
Applications:
Hydrogen production, ammonia synthesis, and IGCC power plants.
3. Oxy-Fuel Combustion
Oxy-fuel combustion involves burning fuels in a mixture of pure oxygen and recycled flue gas instead of air, resulting in flue gas that is primarily CO2 and water vapor.
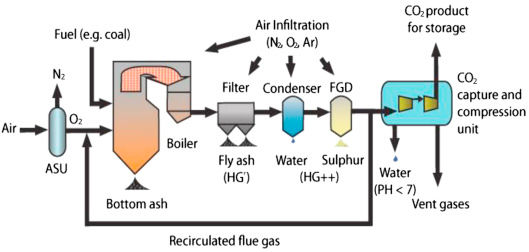
Mechanism:
Combustion in an oxygen-rich environment reduces nitrogen oxide emissions and produces a concentrated CO2 stream.
The water vapor is condensed, leaving a nearly pure CO2 stream for capture.
Advantages:
Produces highly concentrated CO2 streams, reducing the cost of separation.
Enhances thermal efficiency and reduces pollutant emissions.
Simplifies CO2 purification.
Limitations:
High costs associated with oxygen production (via air separation units).
Requires modifications to existing combustion systems.
Limited commercial deployment.
Applications:
Power generation and waste-to-energy plants.
Emerging Carbon Capture Technologies
1. Solid Sorbents
Solid sorbents, such as metal-organic frameworks (MOFs) and zeolites, are gaining attention for their ability to adsorb CO2 under specific conditions.
Mechanism:
CO2 molecules are captured on the surface of the sorbent through physical or chemical interactions.
The sorbent is regenerated by altering temperature or pressure conditions.
Advantages:
High selectivity and adsorption capacity.
Lower energy requirements for regeneration compared to solvents.
Potential for modular deployment.
Limitations:
Limited scalability and durability.
High production costs for advanced sorbents.
Applications:
Industrial gas separation and direct air capture (DAC).
2. Direct Air Capture (DAC)
DAC systems capture CO2 directly from the atmosphere, offering a solution for hard-to-abate emissions.
Mechanism:
Air is passed through a filter or sorbent that captures CO2.
Captured CO2 is released during regeneration and can be stored or utilized.
Advantages:
Addresses dispersed emissions sources.
Can achieve negative emissions when coupled with permanent storage.
Flexible deployment options.
Limitations:
Extremely high energy and cost intensity.
Requires large land areas for deployment.
Still in the early stages of commercialization.
Applications:
Carbon removal initiatives and climate mitigation projects.
3. Membrane Separation
Membrane-based systems use semi-permeable materials to separate CO2 from gas mixtures.
Mechanism:
Gas streams are passed through membranes that allow selective passage of CO2 based on size or solubility differences.
Advantages:
Compact and modular design.
Low energy requirements compared to traditional methods.
Scalability for small to medium-sized facilities.
Limitations:
Limited separation efficiency for low-concentration CO2 streams.
Susceptibility to fouling and degradation.
Applications:
Industrial processes, biogas upgrading, and natural gas sweetening.
Comparative Analysis
Technology | CO2 Concentration | Energy Intensity | Cost | Scalability | Maturity |
Post-Combustion Capture | Low to Moderate | High | High | High | Commercial |
Pre-Combustion Capture | High | Moderate | High | Moderate | Early Commercial |
Oxy-Fuel Combustion | High | Moderate | High | Low | Pilot/Commercial |
Solid Sorbents | Low to Moderate | Low to Moderate | Moderate | Low to Moderate | Emerging |
Direct Air Capture | Very Low | Very High | Very High | Low | Pilot/Emerging |
Membrane Separation | Moderate | Low to Moderate | Moderate | High | Emerging |
Post-combustion capture technology, known for its widespread applicability and compatibility with existing systems, has an average investment cost of $60 per ton of CO2 and an operational cost of $25 per ton of CO2.
Pre-combustion capture, which is particularly suitable for systems involving gasification or reforming processes, incurs a slightly higher investment cost of $75 per ton and operational costs of $30 per ton.
Oxy-fuel combustion, which involves burning fuel in pure oxygen to produce CO2-rich flue gas, requires an investment of $80 per ton and operational expenses of $35 per ton of CO2.
Solid sorbents, a promising emerging technology, present a moderate investment cost of $65 per ton and operational costs of $28 per ton.
Direct air capture (DAC), despite its ability to remove CO2 directly from the atmosphere, is significantly more expensive, with an investment cost of $250 per ton and operational costs reaching $150 per ton.
Membrane Separation technology, which uses selective membranes for CO2 capture, has an investment cost of $70 per ton and operational expenses of $27 per ton of CO2.
The future of carbon capture is brimming with possibilities, but it’s up to us to seize them. As industries race to meet net-zero goals, the question is no longer if carbon capture will play a role—but how fast we can scale it effectively. Are we ready to move beyond pilot projects and embrace full-scale implementation?
Emerging technologies like direct air capture and membrane separation are showing promise, but we must continue investing in research, innovation, and collaboration to make them economically viable. With costs gradually decreasing and regulatory support increasing, we stand at the tipping point of a carbon capture revolution.
The path forward demands bold action—rethinking industrial processes, strengthening policy frameworks, and fostering public acceptance. The choices we make today will define the carbon landscape of tomorrow.
So, let’s ask ourselves: Are we ready to capture the future and shape a sustainable world?
Comments