LNG (Liquified Natural Gas)
- Hüsnü Tolga Eyyuboğlu
- Feb 10
- 10 min read
Updated: Mar 5
Liquefied Natural Gas (LNG) has become a cornerstone in the global energy landscape, offering a versatile and efficient means of storing and transporting natural gas. By cooling natural gas to approximately -162°C (-260°F), it condenses into a liquid form, reducing its volume by about 600 times. This significant volume reduction facilitates the economic and practical movement of natural gas across vast distances, bridging the gap between supply and demand centers worldwide.
1. LNG Production and Storage
The LNG value chain begins with the extraction of natural gas, which is then purified to remove impurities such as water, carbon dioxide, and sulfur compounds. The purified gas undergoes a liquefaction process involving multiple stages of cooling using refrigerants in a cascade cycle until it reaches its liquefaction temperature. This process is energy-intensive, typically consuming about 10-15% of the energy content of the natural gas being processed. The liquefied gas is stored in specially designed cryogenic tanks, often constructed with double walls and insulated with materials like perlite or vacuum spaces to maintain the low temperatures required for LNG stability.
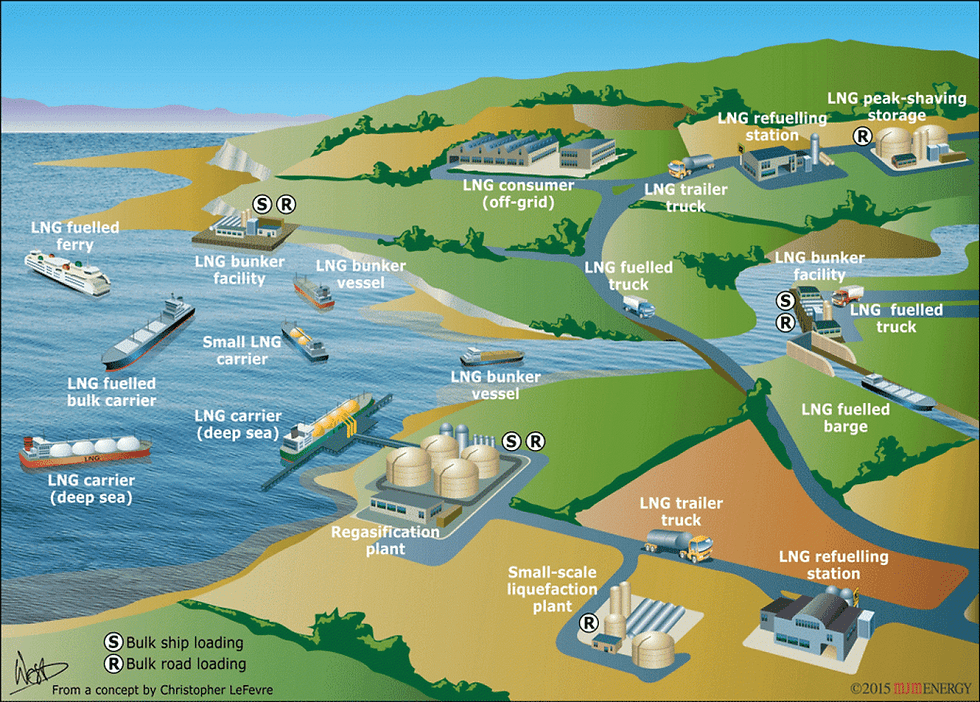
2. Transportation and Regasification
Once liquefied, LNG is loaded onto specialized LNG carriers equipped with insulated tanks that maintain the cryogenic temperatures during transit. These carriers transport LNG to regasification terminals, where it is converted back into its gaseous state through controlled heating. The regasified natural gas is then injected into pipelines for distribution to end-users. The global LNG fleet has expanded significantly, with over 600 vessels in operation as of 2024, reflecting the growing demand for LNG transportation.
3. Applications of LNG
LNG serves multiple roles in the energy sector:
Power Generation: LNG is utilized as a fuel for power plants, providing a cleaner alternative to coal and oil. Natural gas-fired power plants emit approximately 50-60% less carbon dioxide compared to coal-fired plants, contributing to reduced greenhouse gas emissions.
Transportation Fuel: The transportation sector is increasingly adopting LNG as a fuel, particularly for heavy-duty trucks and marine vessels. LNG-fueled ships comply with stringent International Maritime Organization (IMO) regulations on sulfur emissions, offering a pathway to greener shipping.
Industrial Use: Industries such as manufacturing, chemicals, and fertilizers use LNG as a feedstock and energy source due to its high efficiency and lower environmental impact.
4. Economic Considerations
The economics of LNG projects are influenced by several factors:
Capital Expenditure (CapEx): Developing LNG infrastructure, including liquefaction plants, storage facilities, and regasification terminals, requires substantial investment. For instance, the Guangdong Energy Group's LNG terminal in Huizhou, China, which commenced operations in 2024, involved an investment of approximately $1 billion. (Reuters)
Operational Expenditure (OpEx): Operating costs encompass expenses related to energy consumption during liquefaction, maintenance of cryogenic equipment, and transportation logistics. The energy required for liquefaction can account for up to 15% of the total energy content of the gas.
Market Dynamics: LNG prices are subject to global supply and demand dynamics. Factors such as geopolitical events, seasonal demand fluctuations, and competition from alternative energy sources can influence pricing. For example, in late 2024, LNG freight rates experienced a significant decline due to an oversupply of vessels, highlighting the market's volatility. (Financial Times)
5. Best Practices and Lessons Learned
The LNG industry has developed several best practices to enhance safety, efficiency, and environmental performance:
Safety Protocols: Strict safety standards govern the design, construction, and operation of LNG facilities. Implementing robust safety management systems and emergency response plans is crucial to prevent and mitigate incidents. The United Nations Economic Commission for Europe (UNECE) provides comprehensive guidelines on LNG safety and best practices. (Birleşmiş Milletler AEK)
Environmental Stewardship: Minimizing methane emissions throughout the LNG value chain is essential due to methane's high global warming potential. Technologies such as vapor recovery units and leak detection and repair programs are employed to address this issue.
Technological Innovation: Advancements in liquefaction technologies, such as modular and small-scale LNG plants, offer flexibility and reduced costs. The development of Floating Storage Regasification Units (FSRUs) has also enabled faster deployment of LNG infrastructure, as demonstrated by the Alexandroupolis FSRU in Greece, which began operations in 2024. (Reuters)
6. Technology Readiness Level (TRL)
The LNG industry encompasses technologies at various stages of maturity:
Liquefaction and Regasification: Conventional large-scale liquefaction and regasification technologies are well-established, operating at TRL 9, indicating full commercial deployment.
Small-Scale LNG: Small-scale LNG plants, designed for niche markets and remote areas, are at TRL 7-8, with several pilot projects transitioning to commercial operations. A study published in the journal Applied Sciences in 2020 presented a comprehensive procedure for the thermodynamic design and optimization of small-scale LNG production systems, highlighting their potential for broader adoption. (MDPI)
Floating LNG (FLNG): FLNG facilities, which enable offshore gas fields to be developed without the need for onshore infrastructure, are at TRL 8-9. The successful operation of FLNG units, such as Shell's Prelude FLNG, underscores the technological maturity of this approach.
7. Comparison of LNG vs. Pipeline Gas
Liquefied Natural Gas (LNG) and pipeline gas are two major methods of transporting natural gas to meet the growing global demand for energy. Each method has distinct advantages and challenges, depending on the infrastructure, geographical location, and economic factors.
Comparison Metric | LNG | Pipeline Gas | Sources |
Energy Density (MJ/m³) | 23.9 | 37.5 | |
Transportation Cost (USD/MMBtu) | 3.5 | 1.5 | |
Infrastructure Cost (USD Million) | 1,000 | 5,000 | |
Storage Losses (%) | 1.0% | 0.1% | |
Carbon Footprint (gCO2e/kWh) | 0.7 | 0.5 | |
Flexibility (Score) | 8 | 3 |
Key Insights:
Energy Density: Pipeline gas has a higher energy density (37.5 MJ/m³) compared to LNG (23.9 MJ/m³), making it more efficient for stationary applications where pipelines are available.
Transportation Cost: LNG transportation is more expensive at $3.5/MMBtu due to specialized storage and shipping requirements, whereas pipeline gas costs around $1.5/MMBtu, depending on pipeline length and capacity.
Infrastructure Cost: Pipeline infrastructure is significantly more capital-intensive, requiring investments around $5,000 million, while LNG infrastructure, including liquefaction plants and regasification terminals, costs approximately $1,000 million.
Storage Losses: LNG storage involves boil-off losses of about 1%, while pipeline gas storage losses are negligible, at around 0.1%, thanks to underground storage facilities.
Carbon Footprint: LNG's liquefaction and regasification processes contribute to a slightly higher carbon footprint (0.7 gCO2e/kWh) compared to pipeline gas (0.5 gCO2e/kWh), though both are cleaner alternatives to coal and oil.
Flexibility: LNG provides greater flexibility as it can be transported globally via ships, making it ideal for regions lacking pipeline infrastructure, whereas pipeline gas is limited to fixed routes.
LNG infrastructure demands rigorous safety measures to manage its cryogenic properties, while pipeline systems must address challenges related to leaks and pressure regulation. The adoption of Floating Storage Regasification Units (FSRUs) presents a cost-effective and rapid deployment alternative for LNG, enhancing its accessibility and feasibility. While pipeline networks provide stability through consistent demand, LNG offers greater flexibility to adapt to fluctuating market conditions, making it a versatile solution for evolving energy needs.
8. Case Studies
Liquefied Natural Gas (LNG) terminals play a pivotal role in the global energy infrastructure, facilitating the import, export, storage, and regasification of natural gas. Below are six detailed case studies highlighting various LNG terminal projects worldwide, encompassing their capacities, operational specifics, and strategic significance.
Guangdong Energy Group LNG Terminal – Huizhou, China
Initiated in July 2021, the Guangdong Energy Group commenced the construction of a significant LNG import terminal in Huizhou, Guangdong Province. The project, with an estimated investment of approximately $1 billion, is designed to enhance the energy infrastructure in southern China. The terminal features three LNG storage tanks, each with a capacity of 200,000 cubic meters, and a berth capable of accommodating LNG carriers ranging from 80,000 to 266,000 cubic meters. The facility is engineered to handle a maximum annual capacity of 6.1 million tons of LNG. Mechanical completion of the storage tanks was achieved ahead of schedule in January 2024, marking a significant milestone towards operational readiness. Commercial operations are slated to commence by mid-2024, with ExxonMobil securing a 20-year agreement to handle 1.8 million tons of LNG annually through this terminal. (Offshore Energy) (LNG Industry) (PGJ Online)
Tema LNG Terminal – Tema, Ghana
The Tema LNG Terminal represents a landmark project as Sub-Saharan Africa's first LNG regasification terminal. Developed by the Tema LNG Terminal Company, the facility is strategically located at the Port of Tema in the Greater Accra Region of Ghana. Construction commenced in 2018, with operations beginning in March 2021. The terminal comprises a dedicated floating regasification unit (FRU) and a separate floating storage unit (FSU), collectively capable of delivering approximately 1.7 million tons of LNG per annum. The infrastructure includes a 600-meter subsea pipeline and a 6.5-kilometer onshore pipeline, integrating the terminal with Ghana's national gas transmission network. This project is pivotal in diversifying Ghana's energy sources and ensuring a stable supply of natural gas for power generation and industrial use. (AIIM) (ABL Group ASA)
Dragon LNG Terminal – Milford Haven, Wales
Operational since 2009, the Dragon LNG Terminal is situated in Milford Haven, Wales. The facility boasts two LNG storage tanks, each with a capacity of 163,000 cubic meters. In response to demand fluctuations, the terminal is equipped to pressurize, regasify, and deliver natural gas to customers via the National Gas Transmission System (NTS). The strategic location and substantial storage capacity of the Dragon LNG Terminal make it a critical component in the United Kingdom's energy infrastructure, providing flexibility and security in natural gas supply. (Wartsila.com)
Zeebrugge LNG Terminal – Zeebrugge, Belgium
The Zeebrugge LNG Terminal, operated by Fluxys Belgium, is a key hub in Europe's natural gas network. Significant investments have been made to expand its capacity, including the construction of a fifth LNG storage tank to serve a 20-year transshipment contract with Yamal LNG. This expansion underscores the terminal's role in facilitating the import, storage, and transshipment of LNG, thereby enhancing energy security and supply diversification in the region. (TankTerminals)
Dahej LNG Terminal – Gujarat, India
The Dahej LNG Terminal, located in Gujarat, India, is one of the country's largest LNG import facilities. The terminal has undergone several expansions to increase its capacity, reflecting the growing demand for natural gas in India. Studies modeling tidal and wave disturbances, hydrodynamic analyses of breakwaters, and navigation simulations have been integral to optimizing the terminal's design and operations, ensuring efficiency and safety in LNG handling. (Port Technology International)
Stade LNG Terminal – Stade, Germany
Construction of Germany's first land-based LNG import terminal began in Stade, near Hamburg, in 2024. The project, with an estimated cost of around one billion euros, is financed by a consortium of private companies. The terminal is expected to commence operations in 2027, contributing to Germany's strategy to diversify its energy sources and reduce dependence on Russian gas supplies. The development of the Stade LNG Terminal is a significant step in enhancing the country's energy security and transitioning towards a more sustainable energy mix. (Bild)
As the world navigates the complexities of the global energy transition, Liquefied Natural Gas (LNG) emerges as both a solution and a challenge. Its position as a cleaner fossil fuel alternative underscores its potential as a bridge to a carbon-neutral future. However, beneath this promise lies a series of critical questions about LNG’s environmental impact, lifecycle emissions, and long-term sustainability. While LNG’s contributions to reducing coal and oil dependence are undeniable, its true role in the fight against climate change demands deeper scrutiny.
Methane leakage, one of the most pressing concerns in the LNG lifecycle, casts a significant shadow over its environmental claims. Methane, with a global warming potential (GWP) over 25 times that of carbon dioxide over a 100-year period and more than 80 times over 20 years, poses a disproportionate threat to the climate. Even minor leakage rates along the LNG value chain—from extraction and processing to storage and transportation—can erode the emissions benefits of switching from coal to LNG. Current industry estimates suggest methane leakage rates range between 1-2%, yet independent studies indicate the actual rates may be higher, especially in under-regulated regions. Without aggressive action to curb these emissions through advanced monitoring technologies, leak detection and repair programs, and stricter global regulations, LNG risks becoming a false environmental compromise.
Lifecycle emissions further complicate LNG’s environmental narrative. While combustion of LNG is cleaner than that of coal or oil, the energy-intensive liquefaction and regasification processes add a hidden layer of emissions. The liquefaction process alone can consume 10-15% of the natural gas’s energy content, contributing significantly to indirect greenhouse gas emissions. Additionally, the emissions associated with transporting LNG across oceans on specialized carriers and the regasification process at destination terminals add to its carbon footprint. These cumulative impacts question whether LNG truly represents a sustainable energy solution or simply a stopgap in the broader decarbonization effort.
The global warming potential of LNG as a transitional fuel must also be weighed against its ability to adapt to emerging technologies. Innovations such as carbon capture and storage (CCS) integrated with LNG facilities, green hydrogen blending, and hybrid renewable-LNG systems could redefine its role. For example, methane pyrolysis, which splits methane into hydrogen and solid carbon, offers a pathway to utilizing natural gas without releasing CO2. These advancements, however, require significant investments and regulatory support to scale effectively. Without these proactive measures, LNG risks being outpaced by renewables like solar and wind, whose costs continue to plummet while offering near-zero lifecycle emissions.
From a global equity perspective, LNG’s role in addressing energy access is both an opportunity and a challenge. While its flexibility to be shipped anywhere provides energy security to countries without pipeline infrastructure, the high costs of LNG infrastructure and transportation disproportionately burden developing nations. This economic divide further underscores the importance of democratizing access to cleaner energy technologies while minimizing environmental impacts.
In the context of global warming and an urgent need for decarbonization, LNG sits at a crossroads. It offers the flexibility, scalability, and immediate emissions reductions that the world urgently needs, yet its methane leakage, lifecycle emissions, and long-term carbon footprint remain significant barriers. The challenge is not just technological but also systemic, requiring a shift in how LNG is produced, transported, and consumed. Industry players must move beyond short-term profits and commit to transparency, environmental stewardship, and equitable solutions.
Ultimately, LNG must transcend its identity as a transitional fuel and become a catalyst for deeper change. By adopting a lifecycle approach to emissions, aggressively addressing methane leakage, and integrating cutting-edge technologies, LNG can evolve into a cleaner, more sustainable energy solution. However, the responsibility does not rest solely with industry; governments, regulators, and consumers must also demand accountability and support innovation. The question is not whether LNG can bridge the energy gap, but whether it can do so without compromising the very climate it aims to protect. This is LNG’s defining challenge—and its greatest opportunity.
Comments