Life, as We Know It & What Has Carbon Got to Do with It?
- Hüsnü Tolga Eyyuboğlu
- Feb 27
- 11 min read
Updated: Mar 5
Throughout our lives, we've all pondered the age-old question: "Does life exist beyond our planet?" But what we're truly curious about is whether complex, carbon-based life forms inhabit the vast reaches of space, rather than just bacteria or single-celled organisms. We are, to be exact, curious about aliens. The quest for extraterrestrial life has captivated our imaginations for centuries, and while definitive answers still elude us, our understanding of carbon-based life in the cosmos has grown considerably.
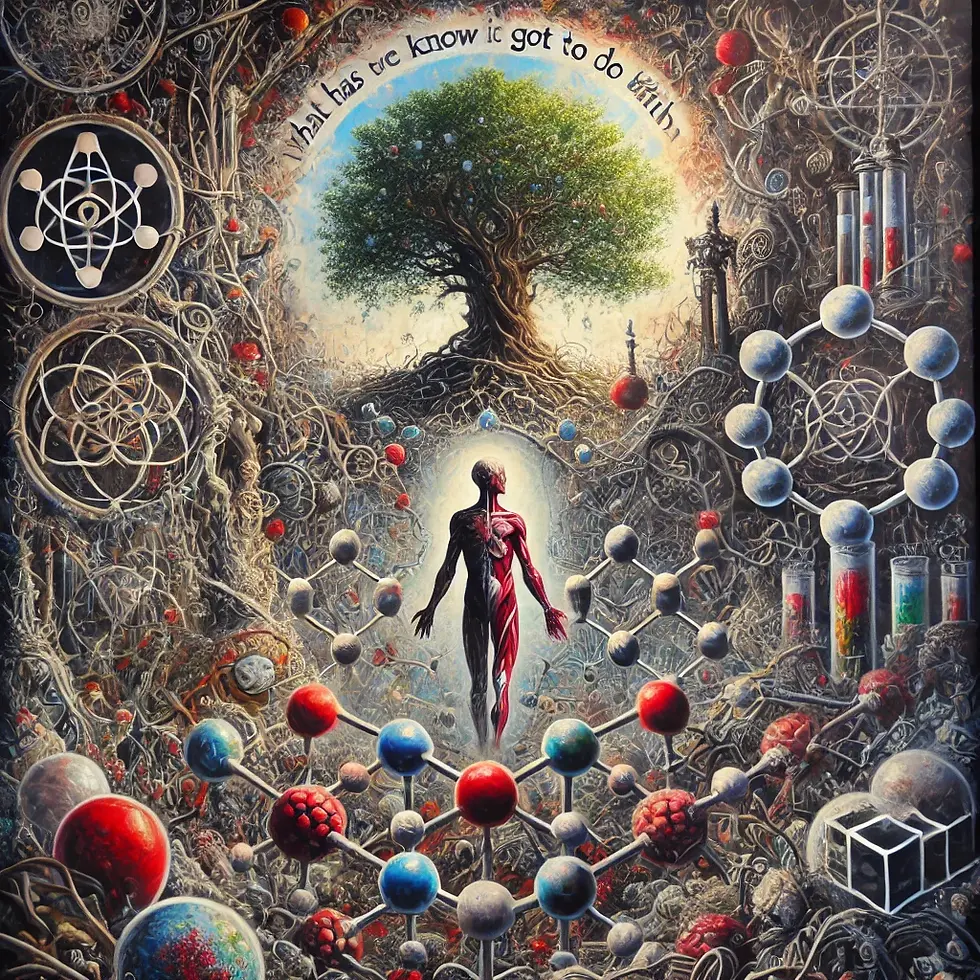
One crucial aspect we've come to appreciate is the necessity of an atmosphere for sustaining carbon-based life. Planets capable of supporting life must possess the ability to maintain a stable mix of gases, creating an environment conducive to complex life forms.
Equally vital is the carbon cycle, the intricate process essential for the development of complex life. The carbon cycle we observe today is the culmination of countless millennia of evolution. Earth, a relatively young planet at 4.6 billion years old, has been home to carbon-based life for approximately 3.5 billion years as we know it today. These ancient inhabitants not only persist but have played a pivotal role in initiating the carbon cycle, gradually oxygenating the atmosphere and shaping the environment we thrive in.
Despite the ever-changing surface conditions of our planet over geological timescales, humanity cherishes the stability of our current environment and strives to preserve it. This instinctual urge to maintain conditions conducive to our survival speaks to a deeper philosophical question about our relationship with the natural world.
The Journey of Carbon: From Life’s Origins to Earth’s Carbon Sinks
Carbon, the essential building block of life as we know it, began its journey billions of years ago in the depths of cosmic explosions. Forged in the nuclear fusion of ancient stars, carbon atoms were scattered across the universe, eventually seeding planets like Earth with the fundamental ingredients for life. But how did this simple element evolve into both the intricate web of biological complexity we see today and the vast carbon sinks that regulate our planet’s climate?
The story begins 4.6 billion years ago, when Earth was a molten, chaotic sphere bombarded by cosmic debris. Intense volcanic activity released vast amounts of carbon dioxide (CO₂), methane (CH₄), and other gases into the primitive atmosphere. As the planet cooled and liquid water bodies formed about 4.4 billion years ago, the oceans became Earth's first major carbon sink, absorbing CO₂ from the atmosphere. This dissolved carbon reacted with seawater, leading to the formation of carbonate minerals, which eventually settled into limestone and sedimentary rock formations, locking carbon away for geological timescales.
Meanwhile, carbon’s remarkable ability to form long, stable chains and complex three-dimensional structures made it the foundation for life’s emergence. Around 4 billion years ago, Earth’s primordial environment—rich in volcanic gases—interacted with energy sources such as lightning, geothermal heat, and ultraviolet radiation, triggering the formation of organic compounds like amino acids, nucleotides, and simple sugars. The famous Miller-Urey experiment (1953) demonstrated that under conditions mimicking early Earth, these simple carbon-based molecules could indeed form the building blocks of life.
Over time, these organic molecules accumulated in Earth’s ancient oceans, forming the primordial soup, where carbon-based self-replicating molecules such as RNA emerged. Scientists believe that RNA served as the first carrier of genetic information, predating DNA, and enabling early molecular evolution. Through natural selection, these primitive systems evolved into protocells, simple cell-like structures enclosed by lipid membranes—laying the foundation for life’s evolution.
By 3.5 billion years ago, the emergence of photosynthetic microbes, such as cyanobacteria, marked a defining shift in Earth's carbon cycle. These organisms absorbed CO₂ from the atmosphere and released oxygen, gradually transforming Earth’s atmosphere while also contributing to organic carbon storage in the biosphere. As microbial life flourished, carbon became trapped in biological material, eventually forming fossil fuels—coal, oil, and natural gas—through millions of years of heat and pressure-driven geological processes.
On land, the expansion of forests and soil-based ecosystems further enhanced Earth's capacity to store carbon. By the Devonian period (~400 million years ago), vast swamp forests had emerged, absorbing atmospheric CO₂ through photosynthesis and locking carbon in biomass and deep soil layers. Wetlands and peatlands evolved as highly effective carbon reservoirs, preventing organic matter from decomposing rapidly and thus retaining carbon over extended geological timescales.
Today, these ancient processes have shaped the modern carbon cycle, forming the global carbon sinks—oceans, forests, soils, living organisms, and fossil fuel reserves—that continue to regulate Earth’s atmospheric balance. However, human-driven emissions have rapidly disrupted this balance, accelerating climate change. Understanding how these sinks formed and function is now critical in ensuring their preservation as we confront the challenges of a warming world. The same carbon that gave rise to life must now be managed wisely, lest it reshape the planet in ways we can no longer control.
A Detailed Breakdown of Major Carbon Sinks Today
Carbon sinks are nature's way of balancing atmospheric carbon dioxide (CO₂) levels and maintaining climate stability. They act as storage systems, absorbing and holding carbon for extended periods. Understanding their significance and how they function can help us make informed decisions about protecting them and mitigating climate change.
Let’s dive deeper into the major carbon sinks—the atmosphere, oceans, terrestrial environments, organic matter, and fossil carbon. Each plays a unique role in regulating the planet's carbon cycle, but they are under increasing stress due to human activities.
Atmospheric Carbon: The Dynamic Balancer
The Earth's atmosphere is often the first thing we think of when discussing carbon emissions. It serves as a vast but delicate sink, constantly interacting with the biosphere and hydrosphere.
Key Features of Atmospheric Carbon:
Storage Capacity: Around 750 gigatons (Gt) of carbon.
Main Components: Carbon dioxide (CO₂), methane (CH₄), and carbon monoxide (CO).
Sources of Carbon Input:
Fossil fuel combustion (cars, factories, power plants)
Deforestation and biomass burning
Industrial processes like cement production
Agricultural practices (livestock methane emissions)
Carbon Flux: How It Moves
Atmospheric carbon does not stay still—it cycles through various processes like:
Photosynthesis: Plants absorb CO₂, converting it to oxygen and organic matter.
Respiration: Animals and plants release CO₂ back into the atmosphere.
Ocean Uptake: Oceans absorb atmospheric CO₂ through surface interactions.
Human Impact on Atmospheric Carbon
Due to human activities, atmospheric CO₂ levels have risen from 280 parts per million (ppm) in pre-industrial times to over 415 ppm today. This drastic increase contributes to global warming, leading to rising temperatures and extreme weather patterns.
What Can Be Done?
Transitioning to renewable energy sources
Reducing fossil fuel consumption
Enhancing reforestation and afforestation efforts
Oceanic Carbon: The Silent Guardian
Oceans are the largest carbon sink on Earth, holding around 39,000 Gt of carbon. They play a crucial role in regulating atmospheric CO₂ and maintaining climate balance.
Mechanisms of Carbon Absorption in Oceans:
Solubility Pump:
Cold ocean waters absorb CO₂ more efficiently.
Warmer water releases CO₂ back into the atmosphere.
Biological Pump:
Phytoplankton absorb CO₂ during photosynthesis.
When phytoplankton die, they sink, taking carbon to the deep ocean.
Physical Circulation:
Ocean currents distribute carbon across different depths and regions.
Challenges Facing Oceanic Carbon Sinks
Ocean Acidification: Excess CO₂ forms carbonic acid, harming marine life.
Temperature Changes: Warmer waters reduce carbon absorption capacity.
Overfishing and Pollution: Disrupts the ocean’s natural carbon cycle.
Solutions for Protecting Oceanic Carbon Sinks
Limiting plastic and chemical pollution
Establishing marine protected areas
Reducing industrial emissions that contribute to acidification
Terrestrial Carbon: The Green Powerhouse
Terrestrial carbon is stored in soil, forests, and wetlands, holding around 3,000 Gt of carbon. These ecosystems act as buffers against climate change by capturing atmospheric CO₂.
Key Terrestrial Carbon Components:
Forests:
Trees and vegetation absorb CO₂ during growth.
Deforestation releases stored carbon back into the atmosphere.
Soil Organic Matter:
Stores carbon through decayed plant material and microorganisms.
Soil erosion and degradation release this carbon.
Peatlands and Wetlands:
Act as long-term carbon storage with minimal decomposition.
Disturbance leads to the rapid release of methane.
Threats to Terrestrial Carbon Storage
Deforestation for agriculture and urbanization.
Unsustainable farming practices leading to soil degradation.
Climate change altering precipitation patterns and increasing wildfires.
How We Can Preserve Terrestrial Carbon Sinks
Adopting regenerative agriculture practices.
Implementing stronger forest conservation policies.
Promoting afforestation and reforestation initiatives.
Organic Carbon: The Cycle of Life
Organic carbon refers to carbon stored within living organisms—plants, animals, and microbes—totaling around 560 Gt. This reservoir is highly dynamic, constantly cycling through the ecosystem.
How Organic Carbon Works
Plants absorb CO₂ from the air and store it as biomass.
Animals consume plants, incorporating carbon into their bodies.
When living organisms die, decomposition releases carbon back to the soil or atmosphere.
Human Impact on Organic Carbon Storage
Modern agricultural practices, deforestation, and mass livestock farming have disrupted the natural balance of organic carbon, reducing biodiversity and ecosystem stability.
Steps to Enhance Organic Carbon Sequestration
Supporting biodiversity conservation efforts.
Reducing excessive livestock farming.
Encouraging composting and organic waste recycling.
Fossil Carbon: The Ancient Reservoir
Fossil carbon is the product of millions of years of geological processes, resulting in vast reserves of coal, oil, and natural gas. Fossil carbon once played a critical role in the carbon cycle before human intervention.
Fossil Carbon Storage Estimates:
Coal: Over 900 Gt
Oil: Around 250 Gt
Natural Gas: Approximately 175 Gt
The Fossil Carbon Dilemma
The extraction and combustion of fossil fuels have released immense quantities of carbon back into the atmosphere, fueling climate change at an unprecedented rate.
Challenges of Fossil Carbon Dependence
Continued reliance on fossil fuels contributes to climate change.
Economic dependence on fossil fuel industries.
Environmental degradation from extraction processes.
Transitioning Away from Fossil Fuels
Investing in renewable energy technologies.
Phasing out subsidies for fossil fuel industries.
Encouraging energy efficiency and conservation.
Carbon sinks are essential components of Earth's delicate balance. Each plays a unique and indispensable role in absorbing excess carbon and maintaining climate equilibrium. However, human activities are rapidly altering their ability to function effectively.
Major Carbon Sinks Summarized
This table effectively summarizes all major carbon sinks covered in the article, providing a clear breakdown of storage capacities, components, and fluxes.
Carbon Sink | Storage Capacity | Main Components | Sources of Carbon Input | Carbon Flux Processes |
Atmosphere | 750 Gt | CO₂, CH₄, CO | Fossil fuel combustion, deforestation, industrial processes, agriculture | Photosynthesis (CO₂ absorption), respiration (CO₂ release), ocean uptake |
Oceans | 39,000 Gt | Dissolved CO₂, carbonic acid, organic carbon | CO₂ absorption from atmosphere, marine organism activity | Solubility pump (CO₂ dissolves in cold waters), biological pump (phytoplankton absorb CO₂), circulation |
Terrestrial Carbon (Forests, Soil, Wetlands) | 3,000 Gt | Organic matter, plant biomass, soil carbon | Photosynthesis, decomposition, soil organic matter | Tree growth (CO₂ sequestration), soil respiration (CO₂ release), land use change (deforestation) |
Organic Carbon (Living Organisms) | 560 Gt | Carbon in plants, animals, microbes | Plant and animal biomass, decomposition | Consumption (carbon transfer in food chains), decomposition (carbon return to soil and atmosphere) |
Fossil Carbon (Coal, Oil, Gas) | Coal: 900 GtOil: 250 GtNatural Gas: 175 Gt | Hydrocarbons (solid, liquid, gas) | Geological processes over millions of years | Combustion (releasing stored carbon as CO₂), extraction (carbon transfer into use) |
The Changing Face of Carbon Sinks Over Time
Carbon sinks act as Earth's natural "carbon banks," absorbing and storing carbon from the atmosphere. Historically, these sinks—namely the atmosphere, oceans, terrestrial ecosystems, and living organisms—have maintained a dynamic equilibrium. However, human-driven activities such as deforestation, industrialization, and fossil fuel combustion have disrupted this balance, leading to excessive CO₂ accumulation.
1. The Atmosphere: A Rising Carbon Reservoir
In pre-industrial times, Earth's atmosphere contained around 280 parts per million (ppm) of CO₂. Today, that number has skyrocketed to 415 ppm, primarily due to:
Burning of fossil fuels (coal, oil, and natural gas)
Deforestation and the loss of vegetation that once absorbed carbon
Industrial processes such as cement production and manufacturing
As atmospheric carbon levels continue to climb, the consequences include rising global temperatures, extreme weather patterns, and ecosystem disruptions. Without immediate intervention, the atmospheric carbon load could push the planet past critical tipping points.
Year | CO₂ Concentration (ppm) | Major Contributing Factors |
1750 (Pre-Industrial) | 280 ppm | Natural carbon cycle, minimal human influence |
1850 | 285 ppm | Early industrialization, minor fossil fuel use |
1950 | 315 ppm | Rapid industrial expansion, deforestation |
2000 | 370 ppm | Fossil fuel reliance, mass deforestation |
2020 | 415 ppm | Energy consumption, transportation, agriculture |
2050 (Optimistic Scenario) | 350 ppm | Adoption of carbon capture, reforestation, energy transition |
2050 (Business-as-Usual Scenario) | 500+ ppm | Continued fossil fuel use, insufficient action |
2. The Oceans: Overburdened and Acidifying
Oceans have long served as a major carbon sink, absorbing 25-35% of human-induced CO₂ emissions. However, this comes at a price—ocean acidification. As CO₂ dissolves in seawater, it forms carbonic acid, which:
Harms marine ecosystems, particularly coral reefs and shellfish
Reduces the ocean's ability to store additional carbon
Alters marine food chains and biodiversity
Warmer ocean temperatures further limit their ability to absorb CO₂, creating a dangerous feedback loop that accelerates climate change.
Year | Ocean Carbon Storage (Gt) | Major Contributing Factors |
1750 (Pre-Industrial) | 37,500 Gt | Natural carbon cycle, minimal human impact |
1850 | 37,800 Gt | Early industrialization, minor fossil fuel emissions |
1950 | 38,500 Gt | Industrial expansion, increasing atmospheric CO₂ absorption |
2000 | 39,000 Gt | Rising CO₂ emissions, increased ocean absorption, minor acidification |
2020 (Current Level) | 39,200 Gt | Peak CO₂ absorption, increasing acidification risks |
2050 (Optimistic Scenario) | 39,500 Gt | Enhanced carbon sequestration efforts, stabilized ocean chemistry |
2050 (Business-as-Usual Scenario) | 38,800 Gt | Reduced CO₂ uptake due to acidification, ecosystem disruptions |
3. Terrestrial Carbon Sinks: The Shrinking Green Powerhouse
Forests, wetlands, and soil ecosystems store vast amounts of carbon. However, human-driven deforestation and land-use changes have significantly diminished their capacity. Consider the following statistics:
Earth has lost 1.3 million square kilometers of forest since 1990.
Soil degradation has released billions of tons of stored carbon into the atmosphere.
Urban expansion and agriculture have drastically reduced natural carbon sinks.
The loss of forests and soil carbon storage capacity means more CO₂ remains in the atmosphere, intensifying the greenhouse effect.
Year | Terrestrial Carbon Storage (Gt) | Major Contributing Factors |
1750 (Pre-Industrial) | 3,200 Gt | Extensive forests, natural carbon cycle, minimal human impact |
1850 | 3,150 Gt | Early deforestation for agriculture and settlement |
1950 | 3,050 Gt | Large-scale deforestation, industrial expansion, land-use change |
2000 | 3,000 Gt | Increased CO₂ emissions, degradation of soil carbon sinks |
2020 (Current Level) | 2,950 Gt | Continued deforestation, land degradation, but also reforestation efforts |
2050 (Optimistic Scenario) | 3,100 Gt | Afforestation, regenerative agriculture, improved carbon sequestration |
2050 (Business-as-Usual Scenario) | 2,800 Gt | Unchecked deforestation, land-use change, declining carbon storage |
4. Living Organisms: A Shift in Biomass Composition
Wildlife once played a crucial role in the carbon cycle by regulating ecosystems. However, due to human expansion and agricultural intensification, global mammalian biomass has shifted drastically.
Below table shows how biodiversity in mammals have shifted over last decades.
Year | Humans (Gt) | Livestock (Gt) | Wild Mammals (Gt) | Major Contributing Factors |
1750 (Pre-Industrial) | 5 Gt | 10 Gt | 50 Gt | Minimal human population, abundant wildlife, small-scale agriculture |
1850 | 10 Gt | 20 Gt | 40 Gt | Early industrialization, expanding livestock farming, deforestation |
1950 | 25 Gt | 50 Gt | 20 Gt | Large-scale industrial farming, rapid human population growth |
2000 | 40 Gt | 80 Gt | 10 Gt | Intensive livestock farming, shrinking wildlife populations |
2020 (Current Level) | 60 Gt | 100 Gt | 4 Gt | Peak livestock numbers, biodiversity decline, urbanization |
2050 (Optimistic Scenario) | 70 Gt | 110 Gt | 5 Gt | Sustainable agriculture, conservation efforts, biodiversity recovery |
2050 (Business-as-Usual Scenario) | 80 Gt | 130 Gt | 2 Gt | Further habitat destruction, uncontrolled industrial farming |
This imbalance not only impacts biodiversity but also reduces the ability of natural ecosystems to sequester carbon effectively.
A Defining Moment for Humanity
For billions of years, carbon has been the silent architect of life, cycling through Earth's atmosphere, oceans, and living organisms, shaping the very foundation of existence. Yet, for the first time in planetary history, a single species—us—has disrupted this delicate balance. We have extracted carbon buried for millions of years, burned it for fleeting energy, and released it at rates far beyond what Earth's natural systems can absorb. The result? A planet gasping for stability, its oceans acidifying, forests shrinking, and climate patterns shifting unpredictably.
But here’s the truth: we are not powerless. The same intelligence that harnessed fire, built civilizations, and reached the stars must now be used to correct the course we have set. The question is no longer whether we are changing the planet—we are. The real question is: Will we act in time to shape the future, or will we let the future be shaped for us?
The choices we make today will echo for generations. Will we redefine industry with sustainable energy? Will we restore forests and soils to their former strength? Will we master carbon capture before it masters us? These are not distant possibilities—they are the crossroads of now. The time for hesitation is over. The era of decisive action has begun.
The Earth does not wait. Neither should we.
Comments